With energy savings, there's intent and then there's plan. Industrial facilities in the United States show a sustained interest in energy management. That's the intent: Reduce overall energy usage or sustain usage but increase the amount produced per kW used.
The plan? Sometimes that's a problem.
In manufacturing, a plan will only stick if it has both the wisdom of experience guiding the vision and the ROI numbers to back up the effort. But in energy, there just isn't the body of research out there for an industrial plant manager to use to set baselines for what "reasonable" energy usage looks like in a manufacturing facility. So, how to assess what portion of current energy usage is reasonable and what is wasteful, or of that wasteful portion, what provides high enough ROI to address?
The ROI under discussion here is the cost per kWh as charged by the utility. Those units carry a different rate depending on time of day and year. Reducing that expense is the savings. The investment is the materials and labor required to change energy consumption. The return is the period of time it takes for the reduced utility bill to pay for the investment. The gravy comes after the expense is paid off.
Returning to the issue of the plan, how then to draw up an ROI estimate when there is no industry standard for reasonable energy usage?
Profiling industrial energy usage
Industrial energy usage varies based on multiple variables:
- plant age
- load type and size
- operational schedule, both hours per week and intensity of loading
- number of workers
- climate
- maintenance philosophy.
The answer is: Don't try to manage every kW consumed by your facility. This is the "wisdom of experience" part of the equation. Divide the facility into the electrical infrastructure and then key systems.
Energy savings start with two basic tactics: (1) general inspection of key systems and (2) targeted data gathering, including logging energy usage at the main service entrances and at the key loads.
Identify how much a system is specified to consume, determine how much it is currently consuming, and identify wasteful practices, either in the hours and type of operation, or in the equipment and system itself. To achieve the savings, the facility must address the waste, either by changes in operation, in maintenance, or in equipment and controls.
Energy components
Before we explain how to trace energy consumption, let's revisit how we define and measure energy.
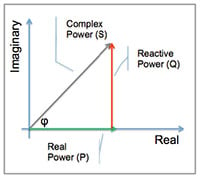
Energy is expressed in real, reactive, and apparent power (Figure 1).
Energy flow is described by:
- real (P) or active power in watts (W)
- reactive power (Q) in volt ampere reactive (VAR)
- complex power (S) in volt ampere (VA)
- apparent power, the magnitude of complex power (VA)
The mathematical relationship of real, reactive, and apparent power can be represented by vectors or expressed using complex numbers, S = P + jQ (where j is the imaginary unit).
Reactive power does not transfer energy - it does not produce work - so it is represented as the imaginary axis of the vector diagram. Real power moves energy, so it is the real axis.
The rate of energy flow in a system is dependent on the load - is it resistive, reactive, or both?
With a purely resistive load, voltage and current reverse polarity at the same time, at every instant the product of voltage and current is positive, and only real power is transferred: work is produced.
If the load is purely reactive, the voltage and current are out of phase, and the product of voltage and current can be positive or negative - indicating some portion of the energy is transferred to the load and some portion flows back. The net transfer of energy to the load is zero: no work is produced.
In reality, all loads have a combination of resistance, inductance, and capacitance, creating both real and reactive power in a system. For that reason, electrical systems are designed to tolerate a certain amount of reactive power. The problem comes when too much reactive power is generated. Not only is there not enough real power to produce the required work, but the overall work-generation capacity of the system is compromised. That's why utilities penalize their customers if their loads produce too much reactive power: it's waste power that costs money to generate but can't be used. Most utility bills track VARs (reactive power), and many calculate power factor, where power factor is a rating of how far below 100 percent real power a system has fallen. Most utilities require their customers to stay above .95 PF (power factor).
Tracing energy consumption
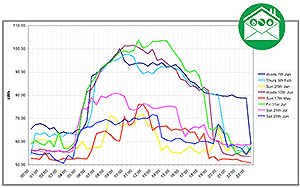
The understanding of basic energy components enables an electrician to set up energy logging equipment to measure overall level and quality of consumption and then trace when energy is consumed by what (Figure 2).
Log power at the main and secondary panels and at major loads. Record kW, kWh, and power factor over a representative period of time.
This provides a very accurate picture of the real power consumption on three-phase circuits and loads.
The biggest power savings come from determining when power usage peaks, evaluating power factor and overall power consumption compared to utility invoices, and possibly rebalancing loads. Even just a couple minutes of peak usage can increase the utility rate for a couple hours, days, or weeks.
Rescheduling loads may allow a company to take advantage of times of day when power is cheaper. Check how far below "1" the power factor is, and check the utility invoices to see if there's a penalty for poor power factor. If there is, the power logger can help to trace the sources. Then, after you've made energy upgrades, reconnect the logger to prove out the benefit of your efficiency improvements.
Understanding energy waste points
Every system and operation has the potential to be a point of waste that can be mitigated or remedied. Electrical subsystems, compressed air, steam, and specific electromechanical systems are a good place to start, but each operation has its own potential waste points that should be measured.
The goal is to map the energy use of specific equipment and processes to look at where energy is being wasted to quantify the waste and prioritize improvements or replacements based on life of the equipment and which modifications can deliver the best return on investment.
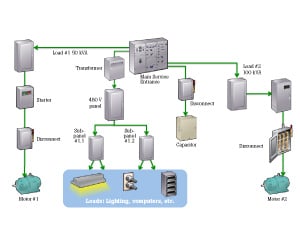
Energy mapping also provides a baseline from which to measure the effectiveness of energy saving projects to justify the cost (Figure 3).
Common wastes in the electrical subsystems:
- Loads commonly left on after hours or unnecessarily run at peak rate time of day.
- No controls on motor may mean more output created than required.
- Over-voltage/current conditions cause excessive power consumption to compensate.
- Phase unbalance causes the load to consume power without being able to use it.
Identify and quantify:
- Thermal scan the electrical panel and mechanical load for overheating.
- Log power over time: How much power is consumed at what time of day and with what amount of waste?
Common wastes and inspection points in electromechanical systems.
- Excess friction from alignment, bearings, imbalance, and looseness overworks the motor, consuming excessive power.
- Uncontrolled loads left on after hours, run at peak rate times, create more output than required, or suffer from over-voltage/current conditions and phase unbalance.
- Aging mechanical devices consume so much more power than new high-efficiency models that early replacement may be justified in reduced kWh consumption alone.
Identify and quantify:
- Thermal scan the drive panel and mechanical load for overheating indicative of electrical inefficiency.
- Log power over time; check total kWh, power factor, peak demand, unbalance, and harmonics.
- Test vibration levels against standards and identify maintenance solutions such as rebalancing.
- Thermally scan couplings/shaft/belts, bearings, and fan.
- Check current and voltage levels.
- Thermally scan termination/junction box and windings, and conduct insulation resistance testing.
Common wastes and inspection points in compressed air systems:
- Excessive leaks in compressed air lines lead to excess operation to maintain supply.
- Compressors left on outside of time of usage waste energy.
Identify and quantify:
- Power log at compressor to baseline consumption.
- Measure pressure at compressor and point of use to determine amount of drop.
- Ultrasound scan lines to identify leaks.
Common wastes and inspection points in steam systems:
- Failed steam traps and insufficient insulation waste steam, causing over-production to maintain necessary supply.
Identify and quantify:
- Power log at boiler to baseline consumption.
- Thermally scan pipes and traps to identify insulation gaps and blockages
Prove the ROI
With the aforementioned lack of industry standards, how do we really know which systems hold the most potential energy ROI? Our best material right now is case examples that embody common situations. Here are several examples for common industrial systems.
Electromechanical inspection
Facility type: steel recycling plant in Germany
Equipment type: belt-driven fan, for process cooling
Measurements taken: vibration testing
Problems noted: moderate unbalance, along with misalignment and bearing wear, was detected.
Savings: Re-balancing was required. A 350 kW motor was running at 80 percent of nominal power; measured power was about 280 kW. After re-balancing, power consumption was reduced by 3 percent. At a cost of 0.11 euro/kWh, annual savings tallied 8,094 euros.
Compressed air inspection
Facility type: manufacturing
Equipment type: compressed air system
Measurements taken: ultrasound inspection of compressed air system (recommended complete data logging of compressor)
Problems noted: the amount of compressed air produced in comparison to the actual demand.
Savings: Multiple savings opportunities were found. Total annual savings of $50,600. Shutting down compressor on weekends: annual savings of $32,700. Install solenoids, to shut air off when machines are shut off: annual savings of $7,100. Repair 36 leaks: annual savings of $4,800. Filters installed in the system at a one-time cost of $6,000; annual savings as a result of the filters: $6,000.
Steam trap inspection
Facility type: manufacturing
Equipment type: boilers and steam lines
Measurements taken: thermal inspection of steam line
Problems noted: six steam traps not operating properly; leaking coils in the plating tanks; steam leaks at plating lines; opportunities to recover condensate
Savings: Six failed traps were replaced at a cost of $500 per trap. Savings achieved: $3,200 per trap using known cost to generate steam and heat loss calculations. Total savings: $16,200.
Next step: Energy log at boiler supply panel before and after addressing leaks and condensate issues.
Increased productivity or reduced overhead?
The next question is a nice one to have to answer: Once you identify a path to reduced energy consumption, do you funnel that savings into increasing the plant yield (same kWh consumption producing greater volumes) or into other business strategies (profit margins, price realization)?
Reducing energy consumption is just good business. By power logging each major system and mapping those costs against utility bills to quantify where and when consumption is occurring, companies can often realize savings by simple operational and schedule changes. By identifying inefficient or outdated equipment, companies can justify and prioritize replacement. And, by reducing overall energy consumption, companies reduce operating costs, improving their competitiveness in the marketplace.
Go to this article in PDF format